Do Smaller Antenna Really Correlate With Higher Resolution? Thorough Examination of One of SAR’s Mysteries Without Using any Formulas!
This time, we are speaking with a broad group of people who are interested in SAR images, including an engineer who processes them, to a business developer, obsessed with the SAR industry, to learn what determines the resolution of SAR images from top to bottom.
Starting Off
Last year was a great year for SAR (synthetic-aperture radar) technology. We wouldn’t be surprised if there are many people keeping an eye out.
The latest news on SAR in particular has everyone talking.
https://spacenews.com/iceye-25-centimeter-resolution/
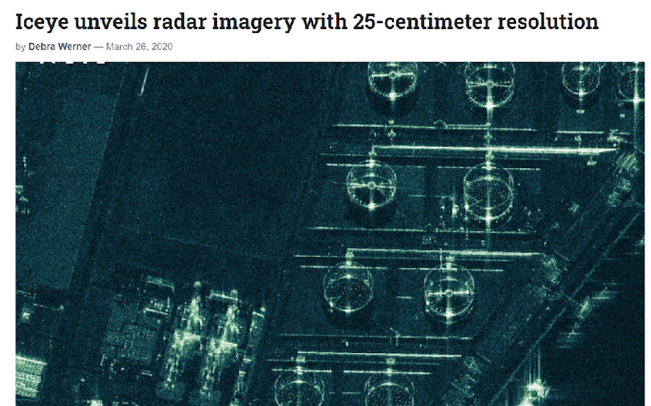
The big news is that SAR has finally achieved a resolution of 25 cm!
The resolution of SAR images is generally considered to be lower than that of an optical satellite due to the wide area it captures, so this news is quite thrilling.
(The fact of the matter is, for Japanese satellites, you don’t come across 25 cm resolution often, even with optical satellites…)
Even more interesting is that the ICEYE satellite isn’t even that big of a satellite, so most people are probably wondering why it can capture images with such a high resolution.
And the reason for that is, smaller antennas actually increase the resolution of SAR images! Who would’ve thought that? “Bigger antennas are definitely better!”, is what most people likely thought up until now just using common sense.
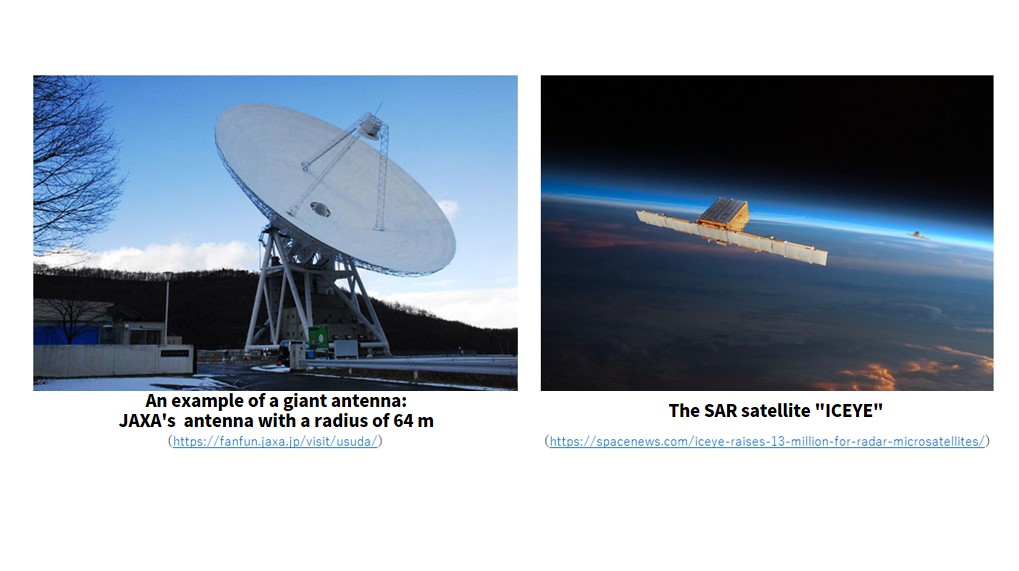
So this time, we decided to speak with a group of people who are interested in SAR images, including an engineer who processes their images, and a business developer who is obsessed with the industry, to learn what determines the resolution in SAR images from top to bottom.
If you read this article, you will be able to explain why smaller antennas lead to higher resolution in SAR images from an engineering standpoint without using any formulas! Maybe you could use it as some fun trivia fact during an outing with friends? In any case, please bear with us as the article gets a little lengthy.
SAR Resolution Is Determined by How Short Its Pulse is
In order to understand the most basic principle behind SAR resolution, we first need to understand what kind of waves are being sent for SAR.
Unlike communication satellites, SAR doesn’t emit a continuous stream of waves over a long period of time.
SAR uses a high output signal called a “pulse”, which is sent out only for short periods of time. Since waves spread out like radiation, they bounce back from the point they hit on the earth’s surface.
When this happens, there are two points on the earth from where these waves bounce back at slightly different timings. When the difference in distance is great enough to detect, these two points are considered to be “resolved”.
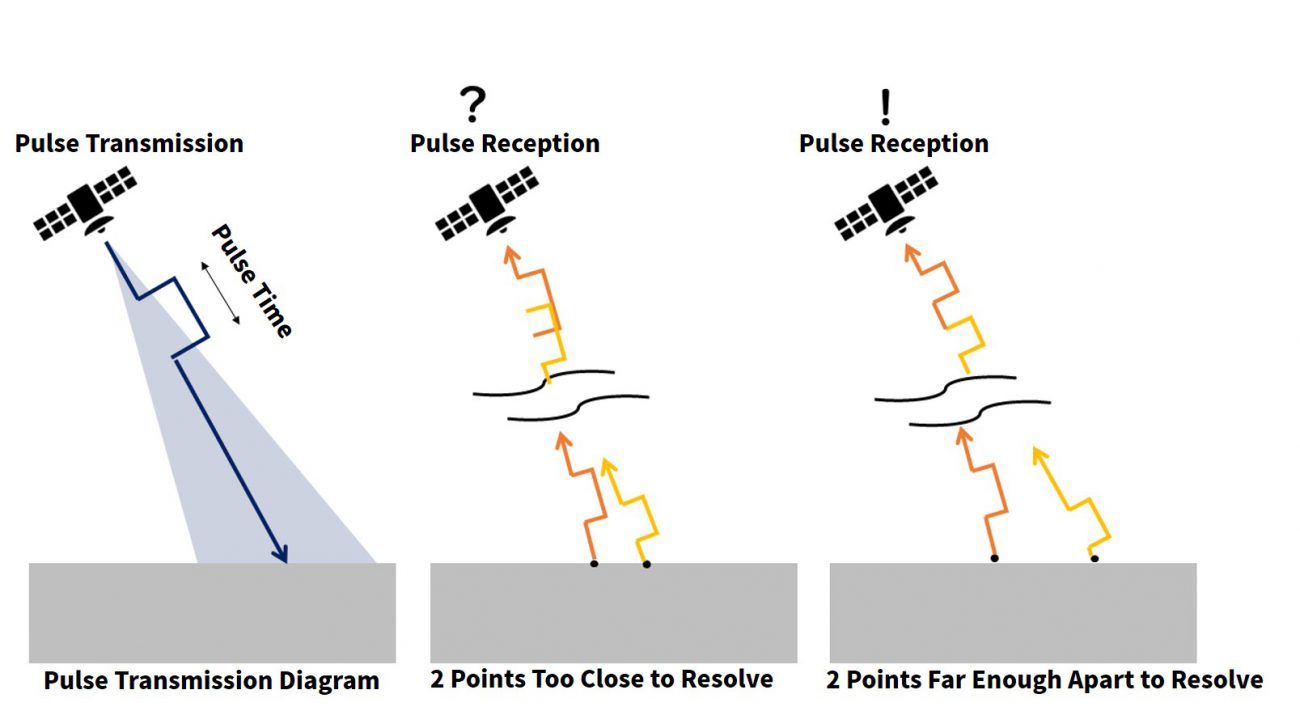
This is the most fundamental way to determine resolution in SAR images.
So what can we do to increase resolution, and increase it even when the two points are close together.
All you have to do is make the pulse as short as possible.
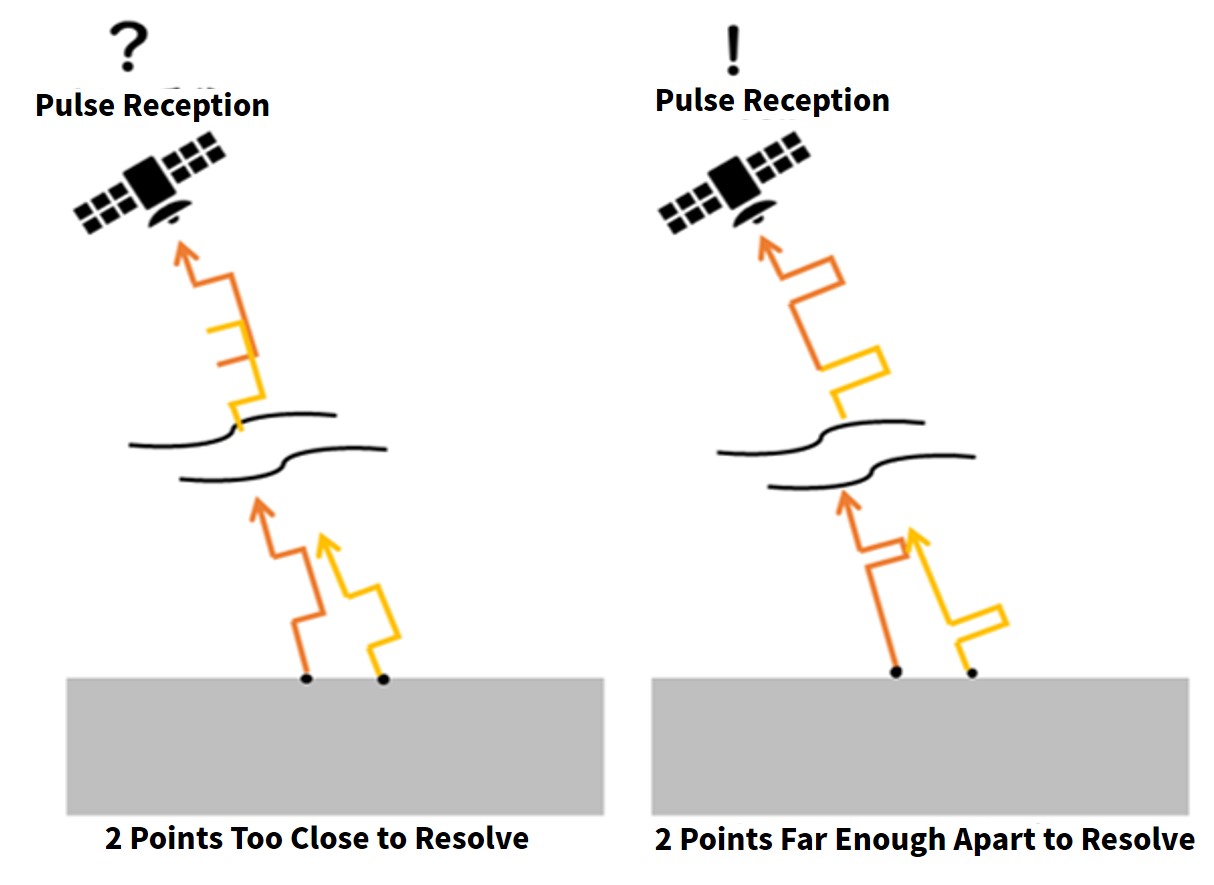
Making a pulse shorter is called “pulse compression”.
The method used to compress SAR pulses is actually kind of ridiculous, so let’s take a minute to break it down.
A Technique to Make Pulses Shorter! "Pulse Compression Technique"
To compress a pulse, you might well guess that you just turn the wave emission on and off really quickly.
The issue with doing that is there is a technical limitation as to how much you can do it. To be more specific, there is a hardware limitation to how quickly electrical currents can be switched on and off.
This is where the pulse compression technique comes into play.
Using the pulse compression technique, we send the pulses with frequency-changing radio waves, unlike the square waves (the ones shaped like squares) we have shown up until now.
The technical term for this is “chirp modulation”.
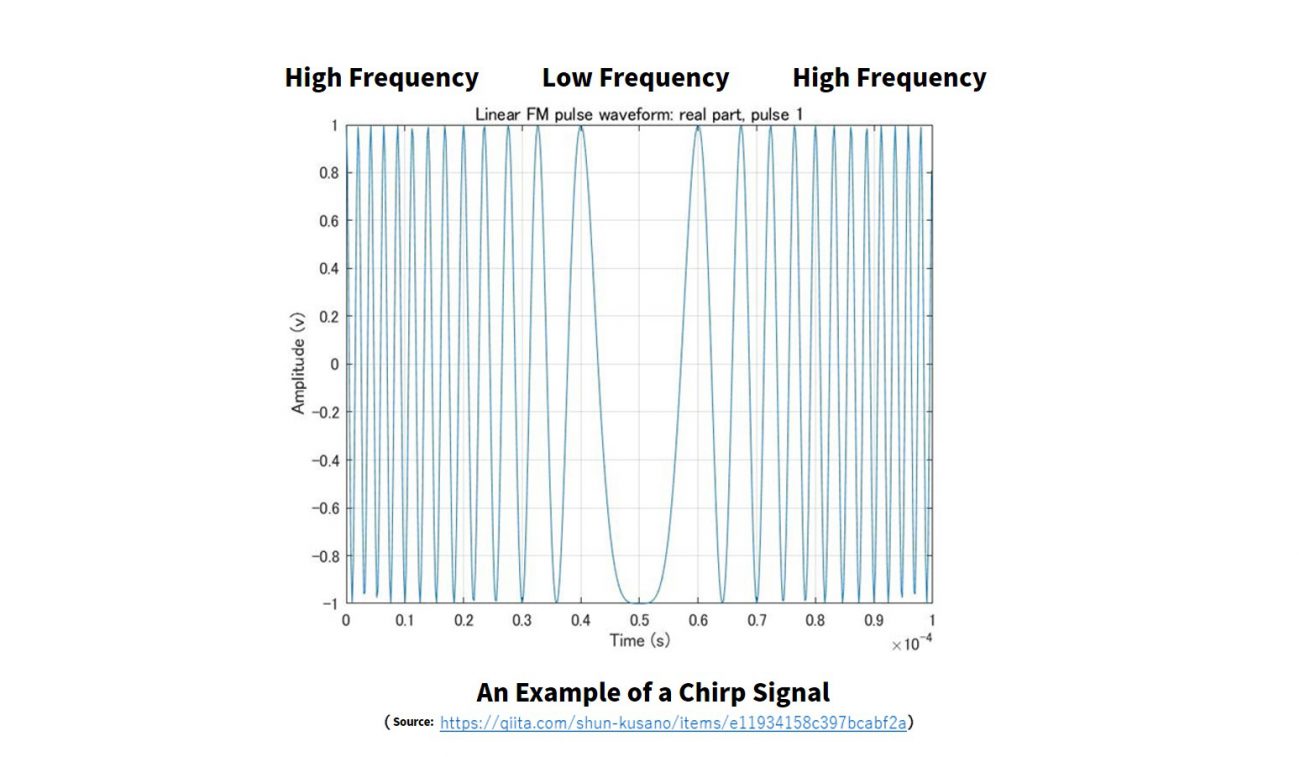
The modulation is the same one used in frequency modulation, or more commonly known as FM, as in FM radio. You listen to different channels on FM radio by changing its frequency.
So what are the benefits of using a chirp signal?
Well, chirp signals can be processed mathematically by what is called a convolution integral to reach its peak at a remarkably quick speed.
What this means is, signals that bounce off the earth’s surface gradually diverge from the original signal that was sent (called the reference signal) and cross-hatch as they return to the sender.
The points where they cross over perfectly create a strong peak, and there is little to no divergence where they don’t cross.
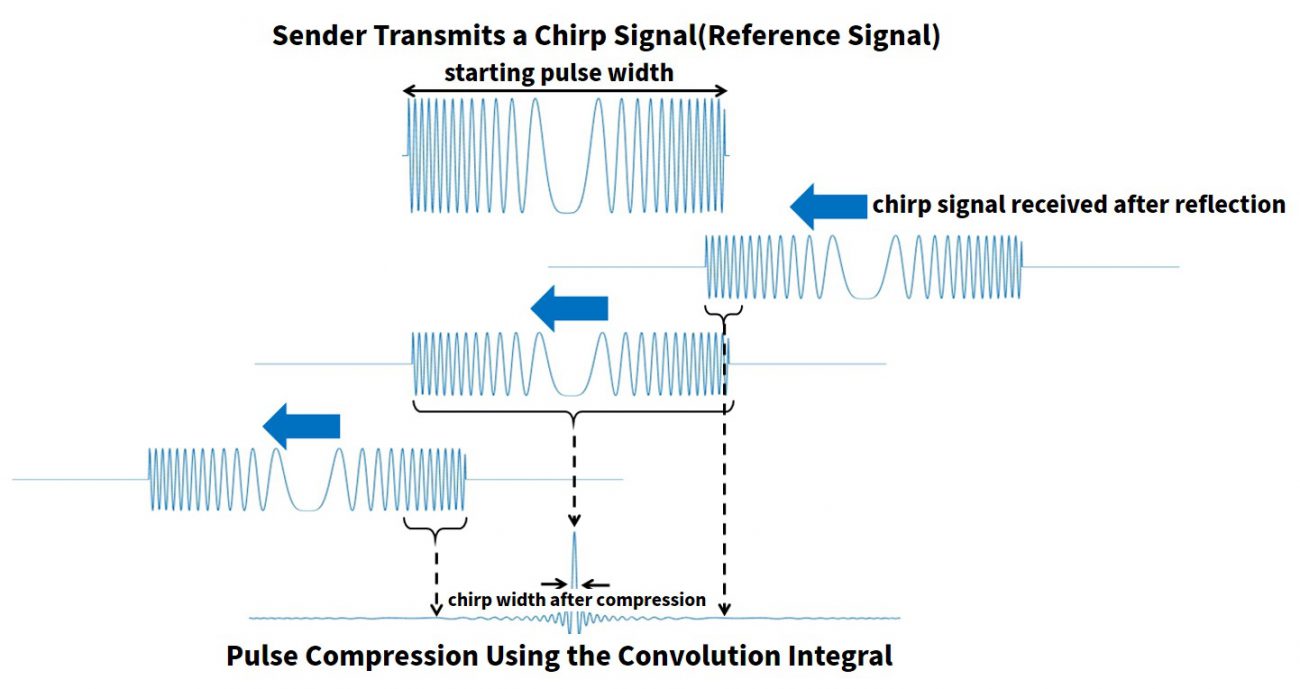
This results in a much more compressed pulse being received, compared to the pulse that was actually sent. You can pick out the waveforms after receiving them, so it is effectively like sending out a wave with a sharp peak.
This is what is called the pulse compression technique.
Incidentally, the convolution integral is mathematically identical to using the Fourier transform and inverse Fourier transform, and is actually used to create images.
Create a Sharper Pulse Broadening the Frequency Spectrum
So now that we understand pulse compression techniques, how can we make our pulses sharper?
The answer is, we need to broaden the frequency spectrum.
A broader frequency spectrum will result in a sharper pulse after performing the convolution integral. (To understand how this works, you need to understand certain mathematical concepts, so we would like to present how this works in a more visual way using the charts below.)
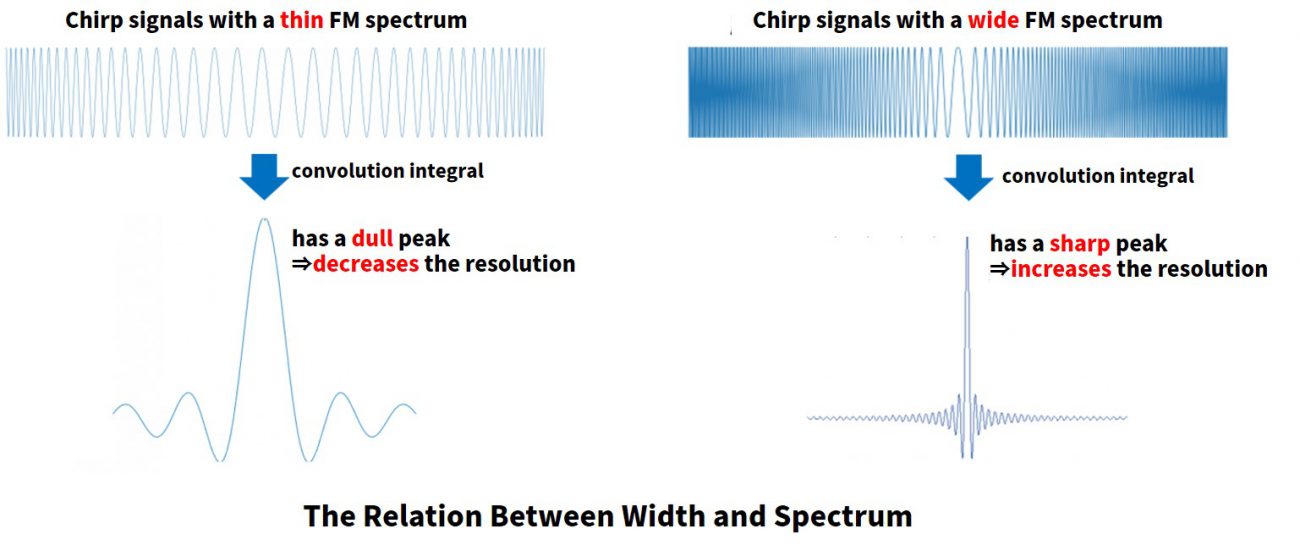
For example: Changing the center frequency set at 1.2 GHz to a spectrum of ±0.2 GHz between 1.0 GHz–1.4 GHz will result in a higher resolution than a spectrum of ±0.1 GHz between 1.1 GHz–1.3 GHz.
So you are probably thinking, why don’t we just make the frequency super broad? Well unfortunately, it doesn’t quite work that way. The frequency spectrum that can be used for SAR is limited by the International Telecommunication Union (ITU).
(First-Half Summary) SAR Resolution Is Closely Tied to the FM Spectrum!
That brings us to the midway point of the battle for understanding the SAR antenna. How do you feel about this? We really do hope you are still with us!
Let’s take a moment to do a quick review:
(1) SAR resolution increases as the pulse signals are sent at short amounts of time.
(2) To compress pulse signals even more, it required a pulse compression technique which uses the convolution integral.
(3) To compress pulses by using the convolution interval, you need to broaden the FM spectrum.
In other words, SAR resolution is closely tied to the FM spectrum! This is what JICA is concerned about.
You may have noticed, but we haven’t even approached the subject of antenna sizes yet.
Don’t worry, this isn’t click-bait! We are going to get into antenna details in the second half.
SAR Resolution Changes Depending on Direction
Now that we understand the pulse compression technique, let’s tread back a bit.
This kind of image will only resolve the direction SAR is facing (called “range direction” below)
This is due to the fact that there is no change in distance between the satellite and the center point along the course on which SAR is moving (called “azimuth direction” below). (Let’s make this simple by thinking that hypothetically, the satellite we are talking about is not in motion. We’ll get into what happens when the satellite moves later on.)
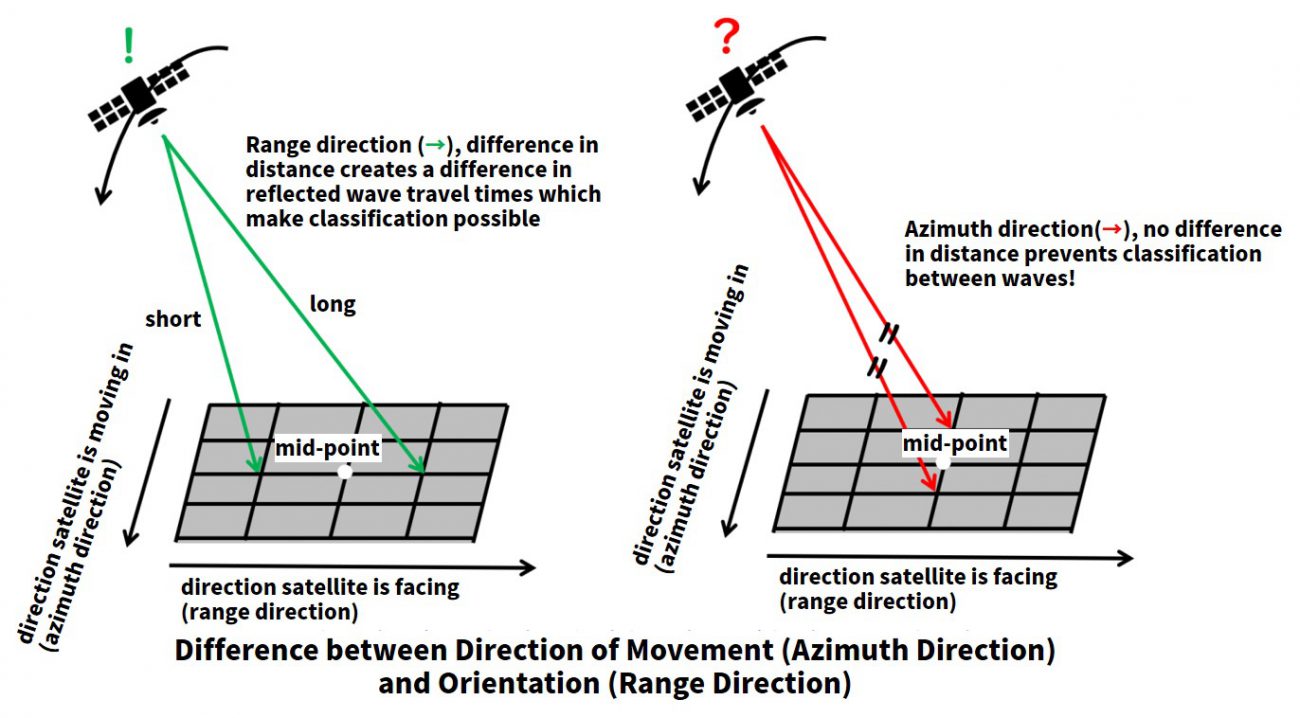
That means that no matter how sharp the pulse is, they can’t be resolved as they come back at the same time.
That means that the only way to increase the resolution after using the pulse compression technique from earlier is to change the range direction!
So how does the azumith direction increase the resolution
It is important to again adjust the frequency. The word we want you to remember is “the Doppler effect”.
Making Chirp Signals With the Doppler Effect!
The Doppler effect is a phenomenon that changes how frequencies appear by moving the source of the signals.
This can be done with sound waves as well.
A good example is when you hear an ambulance siren coming towards you. As the ambulance gets closer, the frequency of its siren shortens, which makes its pitch sound higher. The opposite happens as it moves away from you.
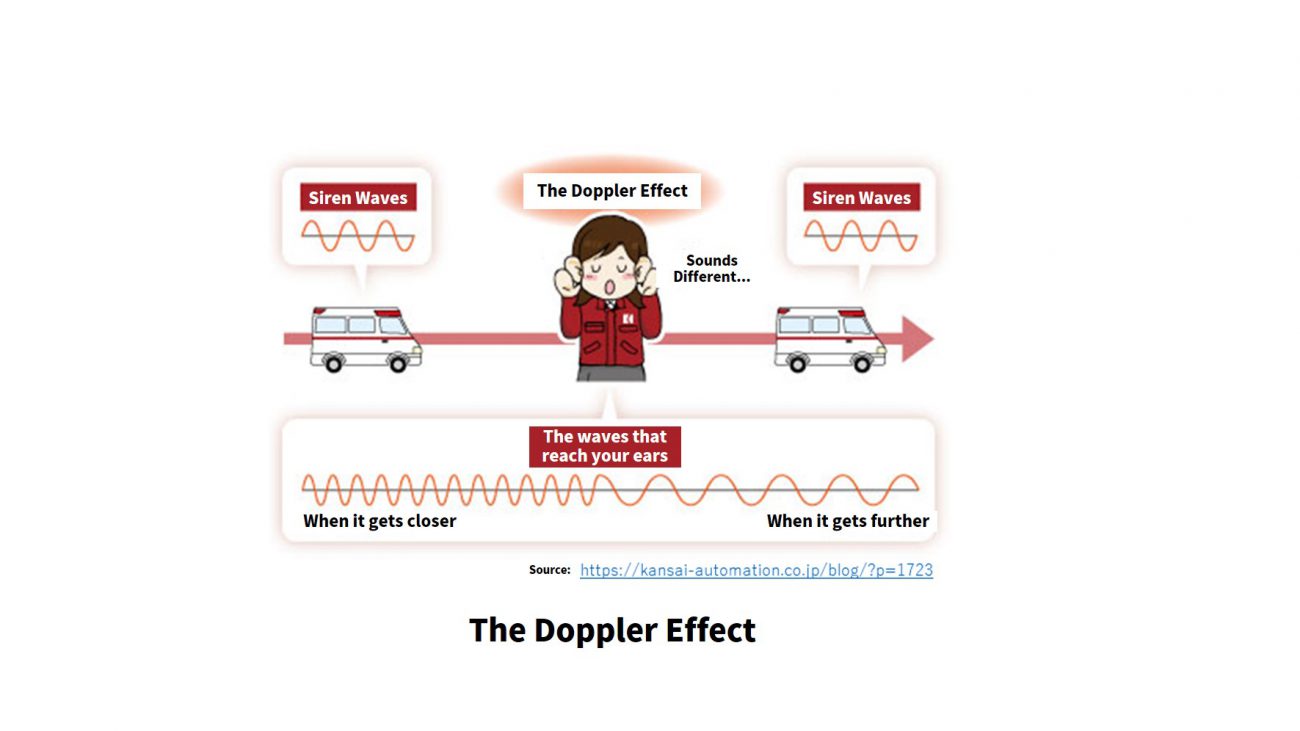
The same exact thing happens with radio waves. Radio waves move at a frequency way higher than sound, but this actually makes it more susceptible to the Doppler effect.
So before we explained that the azimuth direction didn’t change in distance before or after the wave was sent which prevented it from resolving, but the distance doesn’t technically have to change if we use the Doppler effect to bring out differences in the frequency. Let’s figure out how we can resolve the azimuth direction by doing this.
When the satellite is honed in on a target, the chirp signal is sent out at a regular kHz frequency while the radio waves are being emitted. When this happens, as the satellite gets closer to its target, the frequency increases due to the Doppler effect. Conversely, when it moves away from the target, the frequency decreases.
By extracting this adjustment made to the frequency using the Doppler effect (called the Doppler shift), we can create waveforms that are very similar to chirp signals. Through taking this wave and using the convolution integral to compress it creates a pulse with a very sharp peak. This allows us to resolve azimuth direction steered between two points. That is the basic principle behind azimuth direction. By the way, compressing the pulse of an azimuth chirp signal made via the Doppler effect like this is called synthetic aperture processing, and that is where synthetic aperture radars (SAR) get their name.
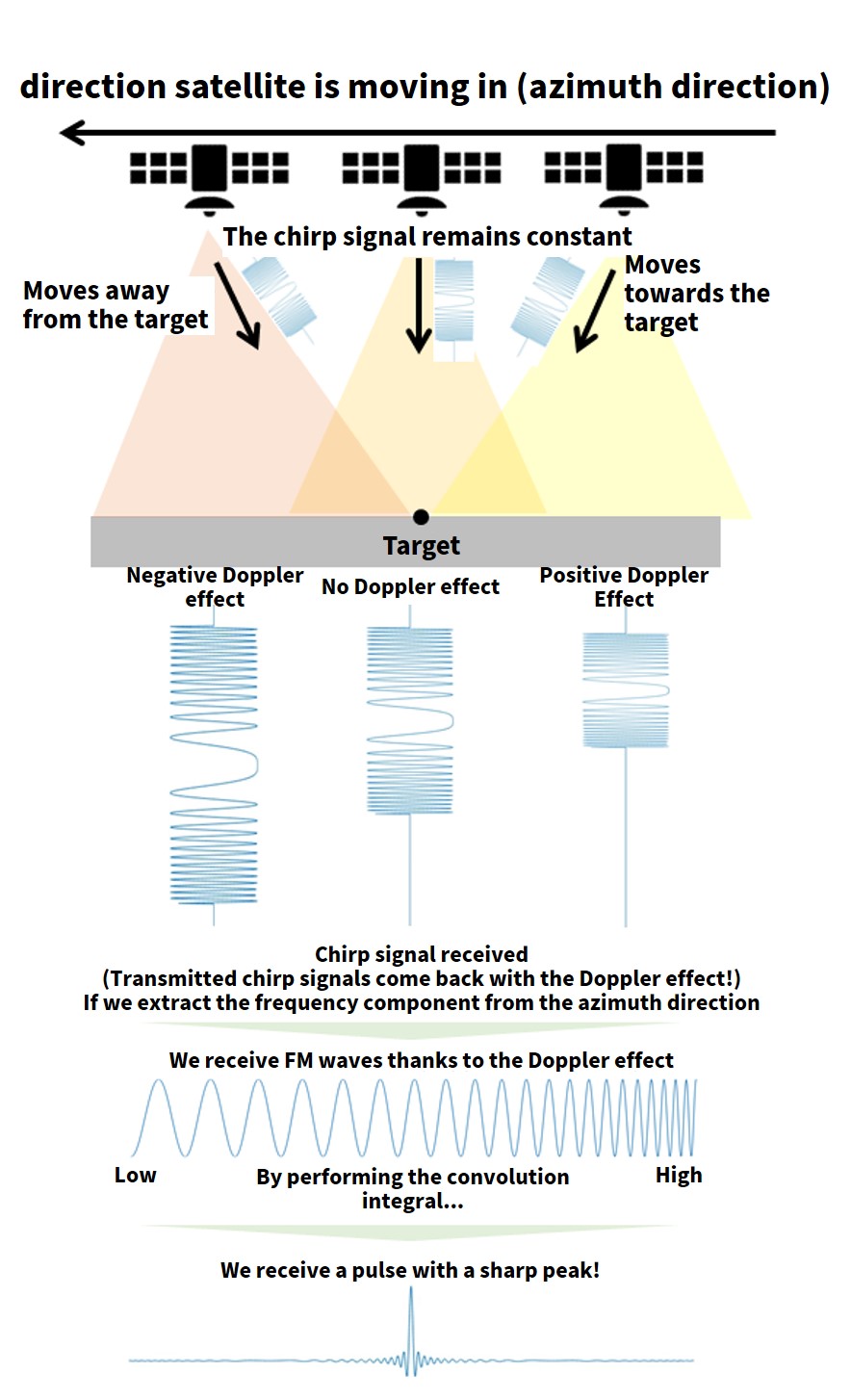
That being said, just like with range direction, the reference waves used for the convolution integral aren’t made by SAR signal components, but are made by considering the trajectory of the satellite, the distance from the earth, and even the earth’s spin to calculate the Doppler effect, so it is actually a very complex process.
In any case, I feel like we may be able to resolve using the azimuth direction now.
So how exactly do we create an even higher resolution?
That’s right! You use the Doppler effect to make the FM spectrum as broad as you can!
Almost There! The Relationship Between Antenna Size and Resolution
Increase the Doppler Effect by Increasing Irradiation Time
Now that we have gotten this far, the final part shouldn’t be too difficult to understand.
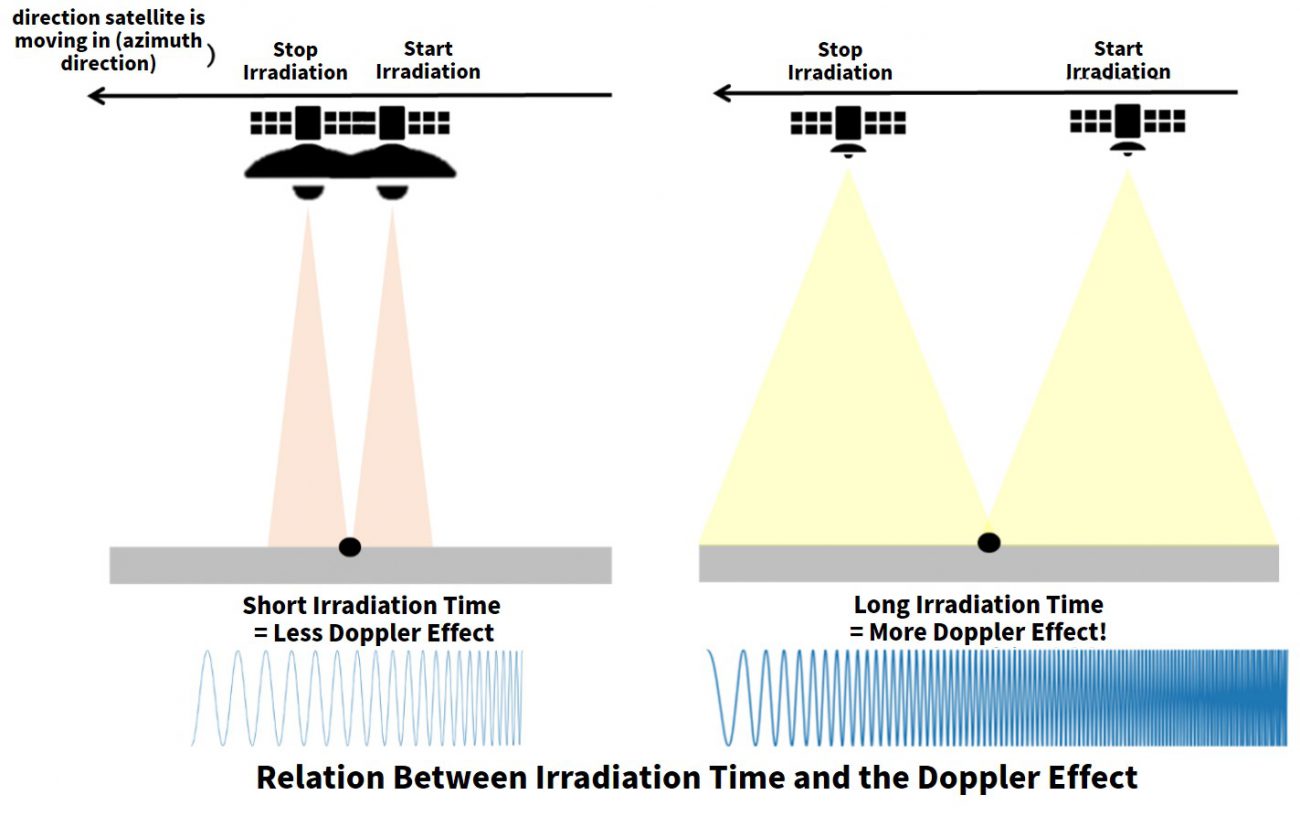
To broaden the FM spectrum using the Doppler effect, you just need to irradiate radio waves for as long as possible, and move from a high frequency spot to a low frequency spot.
Increase Beam Width to Increase Irradiation Time
So how can we make waves irradiate for a longer period of time?
To do this, all we have to do is increase the width of the radar beam (an index that shows how broad waves get) as much as you can!
As the beam gets wider, it allows you to hone in on points that are further away, which means irradiation will take that much longer.
Make Beams Wider by Making Antenna Smaller
The key factor that determines how wide the satellite’s beam will be is the size of its antenna.
Generally speaking, if you use a larger antenna, it will make your beam thinner.
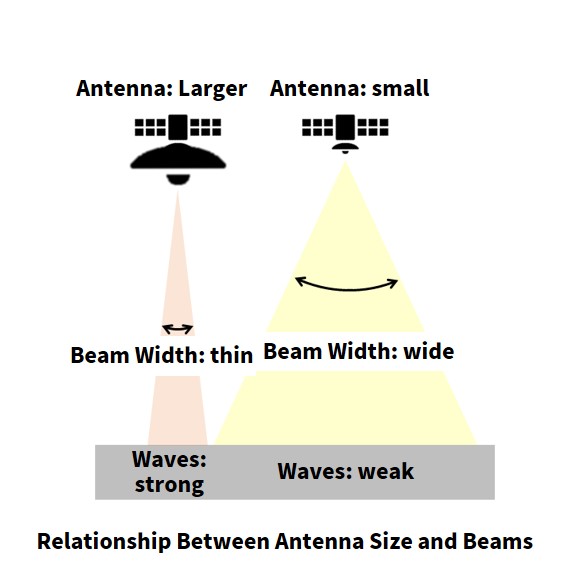
Thinner beams can go longer distances, so in a way it is making your beam capable of sending stronger radio wave outputs.
In many cases, antennas are used to send strong beams to far places, so most people are of the mindset that a bigger antenna must be better.
But for SAR, wide beams work better, so using a small antenna while broadening the beams is important.
By making a small antenna’s beam width broader, you increase the length of time waves are being irradiated, thus increasing the Doppler effect, leading to a more compressed pulse and higher resolution.
That is why a smaller antenna is better for producing higher resolution imagery. And that is our answer to one of the mysteries behind SAR.
We should also mention that if you were to map out the math behind the content of this article, you would come to the surprising result that the azimuth direction resolution is exactly half of the length of the antenna. This doesn’t change no matter what the trajectory of the satellite or its frequency.
For example, the ALOS-2 has a 6 m antenna, and during its generic observation mode (called strip map mode), it records imagery at 3 m resolution, just as the math predicts.
That being said, if you were to increase the width of the beam, the waves would weaken, which would result in a picture with a poor S/N ratio and a lot of noise. This means that you can’t just go and make the antenna as small as possible if you want to gather usable image data.
Spotlight, Another Way to Increase Resolution
By increasing the amount of time the satellite irradiates waves, you can increase the azimuth direction resolution. So how can we increase the resolution even more?
For example, by changing the position of the satellite to hone in on a part of the earth that you want to look at for long periods of time, you can increase the irradiation time.
It is almost as if the satellites are shining a spotlight down on earth at the points they want to watch. (In SAR’s case, the spotlight is a satellite, which is moving at a high speed itself)
The name for this type of observation is incidentally called “spotlight mode”.
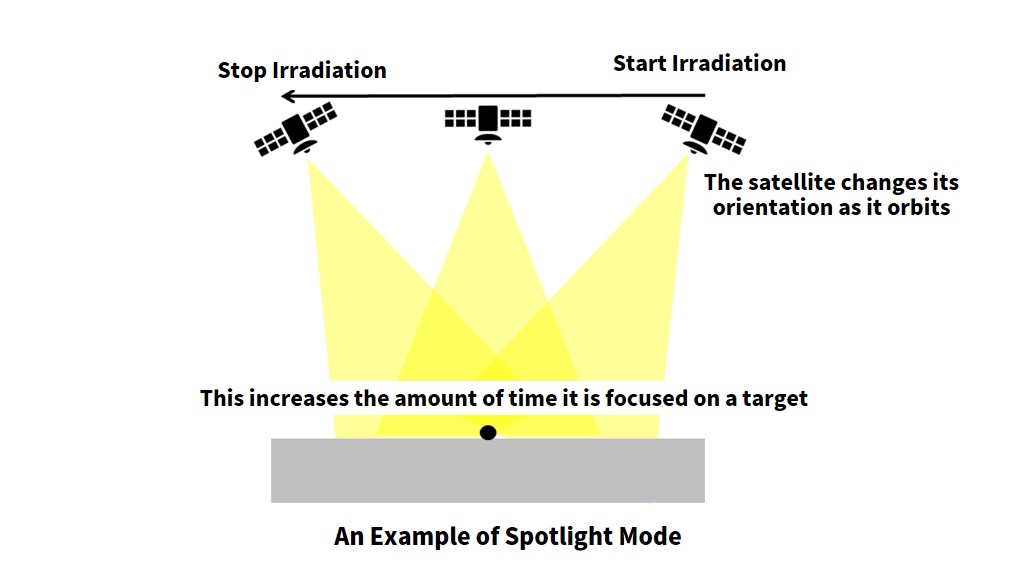
The ALOS-2 uses spotlight mode to increase its azimuth direction resolution by up to 1 m.
The ICEYE with a resolution of 25 cm also uses spotlight mode to up its resolution. It should be noted, however, that this only affects the resolution for azimuth direction. Resolution for range direction is determined only by what we spoke about in the first half; by adjusting its chirp signal spectrum.
And since higher frequencies are affected more by the Doppler effect, L band (set to 1.2 GHz) on the ALOS-2 doesn’t compare to the X band (set to 9 GHz) on the ICEYE when it comes to resolution.
This is how the ICEYE has achieved the world’s highest resolution as a SAR satellite, though the fact that it uses spotlight mode means that the area it observes at any given time is very limited.
In the beginning, we started off by mentioning how generally speaking, the resolution captured by SAR tends to be lower than that of an optical satellite due to the wide area it captures, but through using spotlight mode, it is possible to sacrifice some of its range for more clarity.
More and more Japanese space start-ups are planning to send X band SAR satellites into space, which is exciting news for those to look forward to what kind of products they may bring with them.
Summary
So let’s review what we went over today.
The issue: Do smaller antennas really have a higher resolution?
The answer:
(1) SAR resolution increases as the pulse signals are sent for shorter amounts of time.
(2) To compress pulse signals even more, it required a pulse compression technique which uses the convolution integral.
(3) To compress pulses by using the convolution interval, you need to broaden the FM spectrum.
(4) To broaden the spectrum for the azumith direction, you need to increase irradiation time.
(5) To increase irradiation time, you need to increase the beam width.
(6) A smaller antenna creates a wider beam, so smaller antennas do increase resolution.
Final Thoughts
Thank you for reading up until the very end.
I hope this helped you learn more about how SAR works.
Now that you know a bit more about SAR technology, why not try actually playing around with SAR imagery for yourself?
For those of you who’d like to try, give your hand at analyzing SAR data on Tellus.